Three-dimensional XUV and X imaging.
XUV to X-ray radiation has characteristics that make it particularly attractive for imaging and in particular 3D imaging. On the one hand, the short wavelength (0.01 nm to 50 nm typically) makes it possible to improve the spatial resolution compared to visible imaging by greatly decreasing the diffraction limit. On the other hand, for wavelengths typically less than 1nm, matter begins to become transparent, regardless of its nature. It should be noted that there is a reduced spectral range, between 2.2 and 4.4 nm, for which water is transparent while carbon is still absorbent. This spectral window known as “water” makes it possible to image biological cells with high contrast. The transparency of matter paves the way for non-destructive 3D imaging. Finally, in a manner equivalent to the decrease in the diffraction limit, XUV and X-ray radiation makes it possible to achieve shorter pulse durations than in the visible range, typically attosecond (1 as = 10-18 s) and potentially zeptosecond (1 zs = 10-21 s). With this in mind, for several years now, we have been studying new 3D and ultra-fast XUV and X-ray imager schemes.
XUV Holography and XUV Diffraction: High-order harmonics as well as injected XUV lasers are sources with very high degrees of spatial coherence. High-order harmonics have also demonstrated the possibility of producing attosecond pulses.
In 2006, we performed one of the first holography demonstrations performed with a high-order harmonic bundle [A. S. Morlens et al., Optics Letters, 31, 21, pp. 3095-3097 (2006)]
In 2013, we studied the impact of wavefront defects of the high-order harmonic source on the quality of an XUV diffraction image. [X. Ge et al, Optics Express, 21, 9, pp.11441-11447 (2013)]
In 2015, we performed the first holography experiment with a beam containing a train of attosecond pulses. [G. Williams et al., Optics Letters 40, 13, 3205-3208 (2015)]
X-ray tomography: The reference technique in 3D X-ray imaging is still X-ray tomography, more commonly known as a scanner. Conventional algorithms for processing raw tomography data do not allow the separation of the real part from the imaginary part of the refractive index. However, each of these parts contains specific information about the material being probed. It is therefore interesting to be able to measure them separately
In 2018, we performed X-ray tomography experiments to estimate the distribution of radiosensitizing nanoparticles in healthy and tumor tissues of mice. (fig.1 and 2) [E. Longo et al., Journal of Instrumentation, 13 (2018)] [X. Le Guevel, Nanoscale, 39, 18657-18664 (2018)]
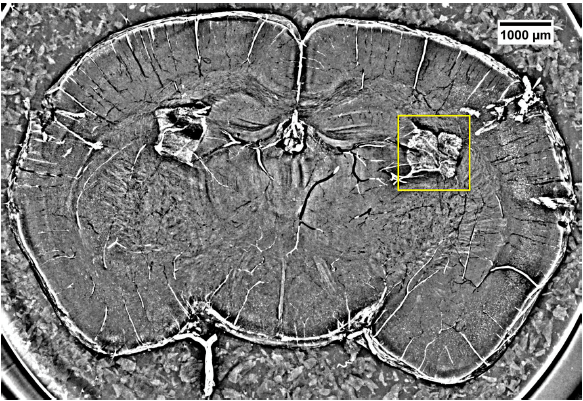
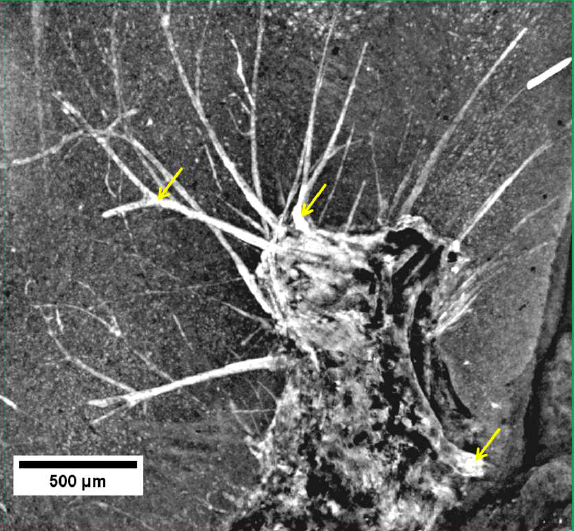
In 2020, we started to numerically and experimentally investigate the possibility of using an X-ray wavefront sensor (Hartmann technique) to measure at the same time but independently the real and imaginary parts of the optical index of the samples. We have shown that it is possible to separate samples by their chemical composition using the two components of the index (fig. 3). Subsequently, we have shown that Hartmann X-ray is much more sensitive than X-ray, allowing, here, to see the berries in a grape seed (fig.4).
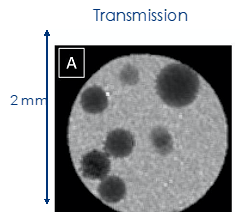
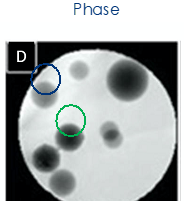
Fig.3: Hartmann X-ray image of a set of spheres of different chemical composition taken at 16 keV. The largest sphere is 0.5 mm, the smallest 0.38 mm. In the transmission image (right), the 4 rightmost spheres appear identical while the phase image shows that they are identical 2 to 2. Subsequent analysis confirmed that the spheres that appear in light gray were made of silicon (blue circle) and the darkest ones were made of Al2O3 (green circle). [ O. de La Rochefoucauld, et al., Nondestructive Testing and Evaluation, 37, 5 p 707-720 (2022)]
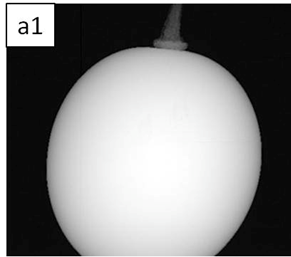
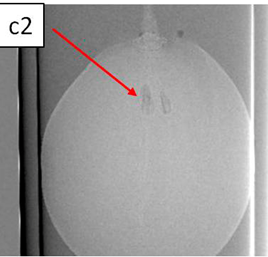
Fig. 4: Images of a grape obtained by absorption (conventional radiography) on the left and by X-ray deflection on the right. In this image, we can see the pips, invisible in X-rays.
XUV and X-ray plenoptics: Today, in X-ray imaging, it is increasingly common to use scanners, also known as X-ray computed tomography (X-ray tomography). The principle of these devices is to record a very large number of 2D images of the same object but acquired from different angles and then to combine them digitally to produce a 3D (3D) image to which various digital techniques can be applied to “see” inside the object without being invasive. X-ray tomography is performed either by rotating the object or, most commonly, by rotating the X-detector source block around the object.
This reference technique has some limitations:
- The quality of the 3D image depends strongly on the number of images acquired in 2D and therefore on the X-ray dose received by the object. In medical imaging, the number of images is limited, but in scientific imaging, the quality of the image is crucial and the dose becomes very high, which can damage the object (see fig. 5)
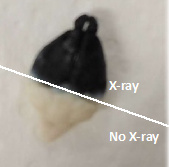
- The production of a large number of 2D images, 3600 in the case of Figure 5, takes time, limiting the possibility of making 3D films.
In 2016, we received European funding from the FET-Open type (VOXEL project) to collaborate with five other laboratories and a company on the transposition of the so-called plenoptic technique from visible to X-rays. Plenoptics consists of coupling a wavefront sensor (in this case a lens array) with an imaging optics in order to make a very large number of thumbnails of the same object in one acquisition. By digital processing, a set of images can be created that are focused at different distances, just as you can physically do using a zoom. From this set, we can reconstruct the object in 3D.
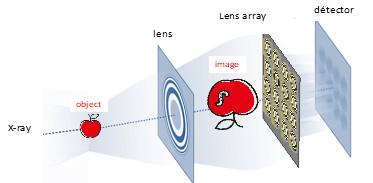
In 2019, we studied contrast variation in digitally refocused images as a classic parameter to evaluate whether an object is in focus or out of frame. [C. Herzog et al, Optics Letters, 2019]. We also performed the first demonstration of an X-ray plenoptic camera (at 11 keV) [E. Longo, et al., MDPI Photonics 9, 2, p98 (2022)].
In 2020, we investigated the correspondence between plenoptics and tomography at limited viewing angles. [N. Vigano et al, J. Imaging, 6, 12 (2020)]. We have also numerically studied the quality of images formed by X-ray Fresnel lenses with very few features [Ying Li, Sensors, 20, 22, 6649 (2020)]. These are very special lenses that are used in the X-lens matrix of the plenoptic camera.
Our current work aims to improve the resolution along the shooting axis in order to get closer to the quality of tomographic images. We work on the development of new plenoptic X-camera architectures as well as on the rendering software.