Quantum sensors

The growing interest in quantum-confined materials is driven by their ability to harness quantum effects at the nanometric scale, paving the way for a new generation of highly sensitive and efficient sensors. In these materials, charge carriers such as electrons and holes are confined within dimensions comparable to their de Broglie wavelength, creating quantized states that radically alter their optical and electronic properties. This quantum confinement enables precise control over the bandgap, light absorption and emission, as well as the fundamental interactions between spins, charge carriers, and the crystal lattice. These characteristics are essential for developing sensors capable of detecting weak signals with unmatched spatial and temporal resolution.
Ultrafast spectroscopy is a key tool for studying and exploiting these quantum-confined materials. This technique allows us to track charge carrier and spin dynamics in real time on ultrashort timescales, on the order of femtoseconds. By probing electronic transitions and interactions within these materials, ultrafast spectroscopy reveals crucial information about the fundamental mechanisms that govern their behavior, such as electron-hole recombination, energy relaxation processes, and spin-orbit coupling effects. This knowledge is indispensable for optimizing the performance of quantum sensors and designing more efficient devices.
Among quantum-confined materials, HgTe (mercury telluride) quantum dots and NV (nitrogen-vacancy) centers in diamond are particularly promising for the development of quantum sensors. HgTe quantum dots, used in LEDs and infrared sensors, stand out due to their high quantum efficiency and their ability to tune the bandgap based on the size and composition of the dots. These features enable the design of high-performance infrared devices for a variety of applications, including remote sensing, medical imaging, and telecommunications, by optimizing electronic transitions in the mid- and far-infrared regions. Their integration into semiconductor devices also holds promise for new ultrasensitive infrared sensors capable of operating at room temperature and accurately detecting weak signals.
On the other hand, NV centers in diamond offer a unique platform for quantum magnetometry. These point defects, formed by a carbon vacancy near a nitrogen atom, exhibit a spin-orbit interaction that can be manipulated by optical and magnetic fields, allowing for ultra-sensitive detection of magnetic fields at the nanoscale. The robustness of NV centers at room temperature and their optical response in the visible spectrum make them particularly attractive for a wide range of applications, from detecting weak biological signals to characterizing quantum materials and advanced electronic devices.
By combining quantum confinement and ultrafast spectroscopy, our research aims to deepen the understanding of these innovative materials, maximize their performance for sensing and imaging applications, and push the current limits of quantum sensors.
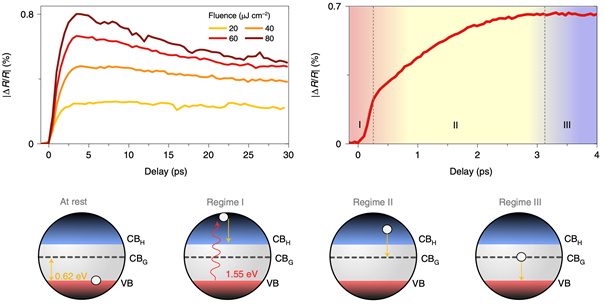
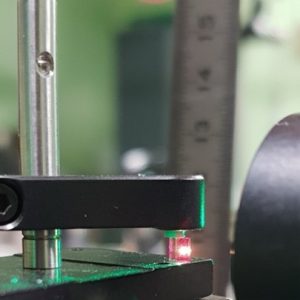
References :
– J. Qu, M. Weis, E. Izquierdo, S. G. Mizrahi, A. Chu, C. Dabard, C. Gréboval, E. Bossavit, Y. Prado, E. Péronne, S. Ithurria, G. Patriarche, M. G. Silly, G. Vincent, D. Boschetto, E. Lhuillier, « Electroluminescence from Nanocrystals above 2 µm », Nature Photonics 16, 38 (2021).
– A. Khalili, M. Weis, S. G. Mizrahi, A. Chu, T. H. Dang, C. Abadie, C. Gréboval, C. Dabard, Y. Prado, X. Z. Xu, E. Péronne, C. Livache, S. Ithurria, G. Patriarche, J. Ramade, G. Vincent, D. Boschetto, E. Lhuillier, « Guided-mode resonator coupled with nanocrystal intraband absorption », ACS Photonics 9, 985 (2022).
Contact : Davide Boschetto
People involved : Amélie Kies, Marie Cherasse, Mateusz Weis, Emmanuel Péronne et Davide Boschetto